Introduction
Climate change is increasingly being recognized for its potential to adversely affect public health. One such consequence of climate change that has been described in the literature is a change in the distribution and habitat of mosquito vectors that are known to transmit disease. The recent emergence of Zika virus and the reemergence of dengue and chikungunya virus in Central and South America have caused increased concern with respect to the effects of climate change on transmission of vector-borne diseases in Canada. Although the mosquito vectors that transmit these common arboviruses are not known to inhabit Canada, it is anticipated that climate change will increase the geographic range of these vectors (1), thereby potentially putting Canadians at risk for the diseases they carry. Furthermore, with the current levels of travel and commerce and the lack of vaccines and effective medical treatment for many of these vector-borne diseases, public health practitioners will be required to anticipate and prepare for the predicted effects of climate change on vector distribution.
Mosquito lifecycles, breeding habits, and feeding behaviours depend on temperature, precipitation, and land use. The United Nations Intergovernmental Panel on Climate Change (IPCC) has predicted an average global temperature increase of 2°C to 6°C between 1990 and 2100 (2). As minimum temperatures are increasing disproportionately to average and maximum temperatures (2), the geographic range of climatic suitability for mosquito vectors of disease will increase, leaving larger populations vulnerable to vector-borne diseases (3). For example, since 2004, there have been two outbreaks of autochthonous dengue in the continental United States: one in Texas (2004-2005) (4) and a second in Florida (2009-2011) (5). More recently, since first being detected in Brazil in 2015, Zika virus has spread across the Americas, with most countries in Latin America and the Caribbean reporting local transmission (6).
The intent of this paper is provide a short review of the available literature to inform public health practitioners and planners of the anticipated effects of climate change on select mosquito-borne disease in humans. This paper is not intended to be a systematic review, but rather to provide an overview of the current literature and summary of anticipated trends. The MeSH term “climate change” was combined with “aedes”, “culex”, or “disease vector” to identify articles for review. To optimize relevance to Canadian practitioners, emphasis was placed on articles that focused on anticipated trends in the Americas.
Mosquito-borne Disease in the Americas
Three mosquitos which have been shown to serve as vectors in the spread of arboviruses are Aedes albopictus, Aedes aegypti, and Culex species. The public health implications of altered vector distribution have most recently been seen in the Americas with the spread of Dengue virus, the emergence of Zika virus, and the reemergence of chickungunya virus. (A number of other less common viruses known to be transmitted by mosquitoes are listed in Table 2 at the end of this paper.)
Aedes albopictus
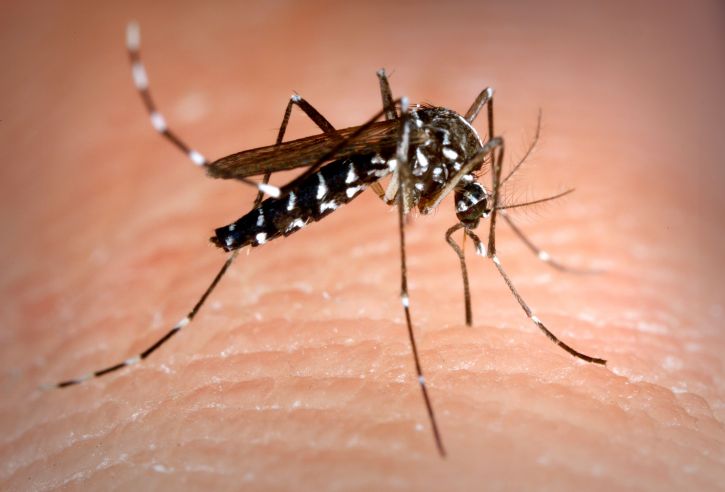
Aedes albopictus, commonly known as the Asian tiger mosquito, has been documented to be one of the fastest spreading species over the past two decades (7). The native habitat of Aedes albopictus is in Asia, but through global trade it has spread to all continents with the exception of Antarctica. In laboratory studies it has been shown to be a competent vector for a number of diseases (Table 1). It is has been implicated in the re-emergence of dengue in France and Croatia (8), and chikungunya in France (9) and Northern Italy (10). Since 2013, autochthonous transmission of chikungunya has been noted in areas of the Caribbean, Latin America, and the United States (11). It is also known to act as a bridge vector of West Nile Virus (WNV), as it feeds on a wide range of hosts, including birds (a competent host) and humans (an incompetent host).
Table 1. Vector-borne viruses and associated diseases transmitted by Aedes albopictus |
---|
Flavivirus • Dengue • West Nile Virus • Japanese Encephalitis Bunyavirus • Jamestown Canyon • Keystone • LaCrosse • Potosi • Cache Valley • Tensaw Alphavirus • Eastern Equine Encephalitis |
Aedes albopictus prefers sheltered containers, whether natural or artificial, for breeding where other resources such as water, food and physical protection may be provided; thus they are categorized as “container-breeding” insects (12). Its spread has been linked to the international tire trade (7) and exportation of “lucky bamboo” plants (13). Its preference for container breeding is an important characteristic that causes it to be commonly associated with urban environments where artificial containers, such as water-storage tanks, tires and bottles, are readily available (14). Furthermore, its capacity to feed on a wide range of host species increases its potential as a vector of disease (15), for example horses in the case of Venezuelan Equine Encephalitis and pigs in the case of Japanese encephalitis (16). In addition, its tendency for day-time feeding greatly enhances its capacity as a vector (17).
A number of studies employed variables such as predicted annual precipitation, annual average temperature, minimum winter temperatures, maximum summer temperatures, and relative humidity in mathematical models to estimate the global range of climatic suitability for Aedes albopictus (14,15,18,19). These studies suggest that, regardless of the CO2 emission levels used in the models, the geographic range suitable for Aedes albopictus can be expected to increase in short, medium, and long-range scenarios (14,15,18,19). Specifically, for North America, studies predict a three-fold increase in the climatically suitable territory in the northeastern United States between 2010 and 2039 (14). Predicted areas of establishment for this mosquito extend as far north as the south shore of Lake Erie and Buffalo, NY and continue to expand northeastward in subsequent years (14), and will likely reach the southern borders of two Canadian provinces, Ontario and Quebec.
In a Canadian study by Ogden et al. (15) three climatic indicators impacting Aedes albopictus survival under current and predicted future climate were analyzed: 1) overwintering (OW); 2) overwintering combined with air temperature (OWAT); and 3) sigmoidal function for linear index of precipitation and air temperature (SIG). These helped determine regions that provide the best conditions for establishment of Aedes albopictus populations in United States and Canada. In this study too, the most extreme models predicted current climatic suitability for Aedes albopictus in southern coastal British Columbia as well as southern Ontario, Quebec, and the Maritimes. Even the most conservative models predicted suitable climate for the species as far north as coastal British Columbia, southern Ontario, Quebec and the Maritimes In the years 2011–2040 (15). However, the authors suggest that in urban and suburban areas that offer refuge from climatic extremes, including very low mid-winter temperatures, expansion may occur much further north than predicted by these models. Geographic distribution of the current and projected climatic suitability for Aedes albopictus are presented in Figures 1 and 2, respectively.
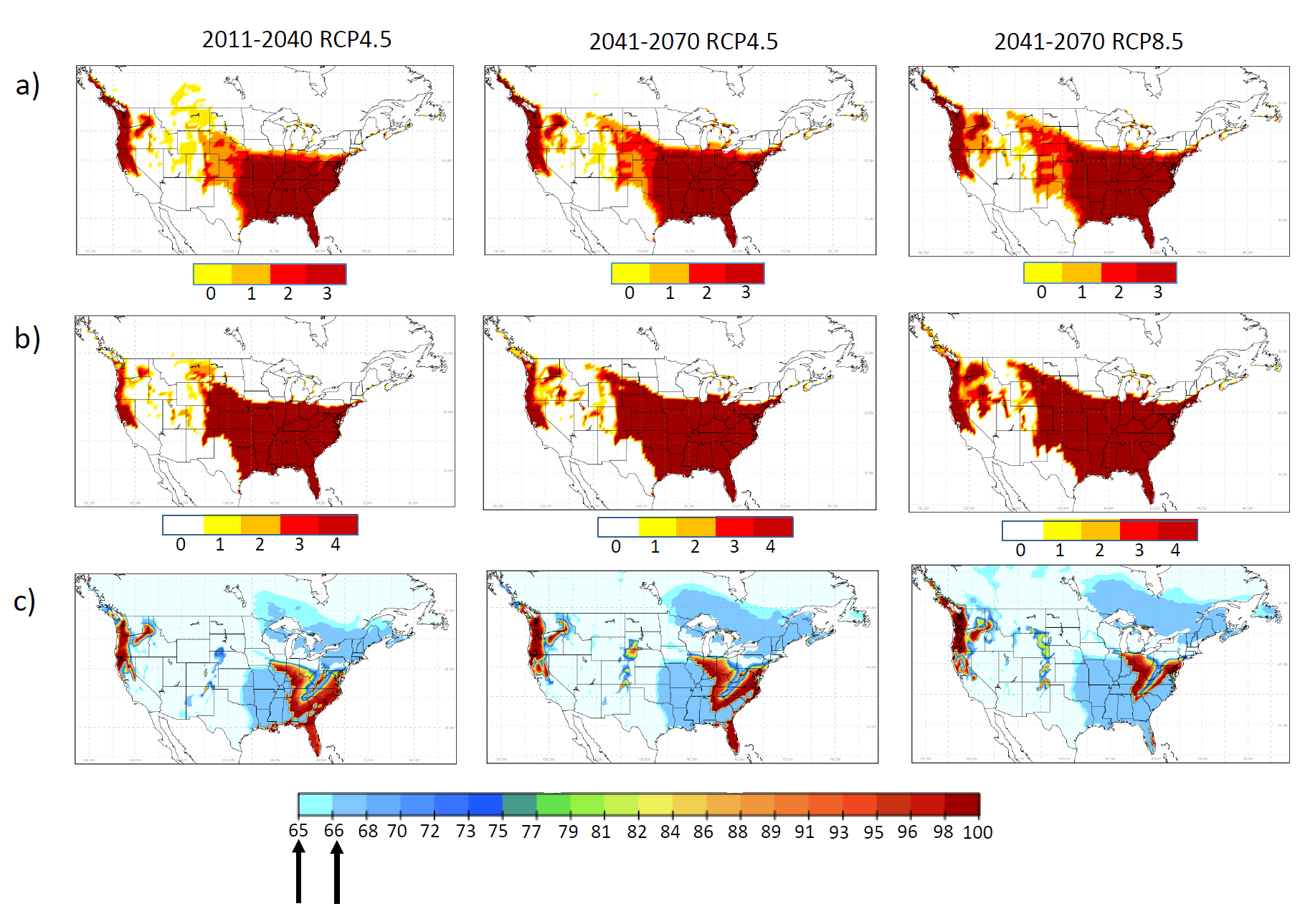
Figure 1: Current climate suitability prediction for Ae. albopictus: a, b, c demonstrate suitability maps of OW, OWAT and SIG indicators for Aedes albopictus from observed climate data between 1981-2010 (left) and CanRCM model output for 1989-2010 (right). For OW and OWAT the color scales correspond to the suitability scale for these indicators (low to high suitability shown from white or yellow to orange and red based on temperature and precipitation values). For SIG the arrows indicate the 64.64% and 66.69% suitability cut-off values. Used with permission from Ogden et al. 2014. Parasites and Vectors, 7:532 (15).
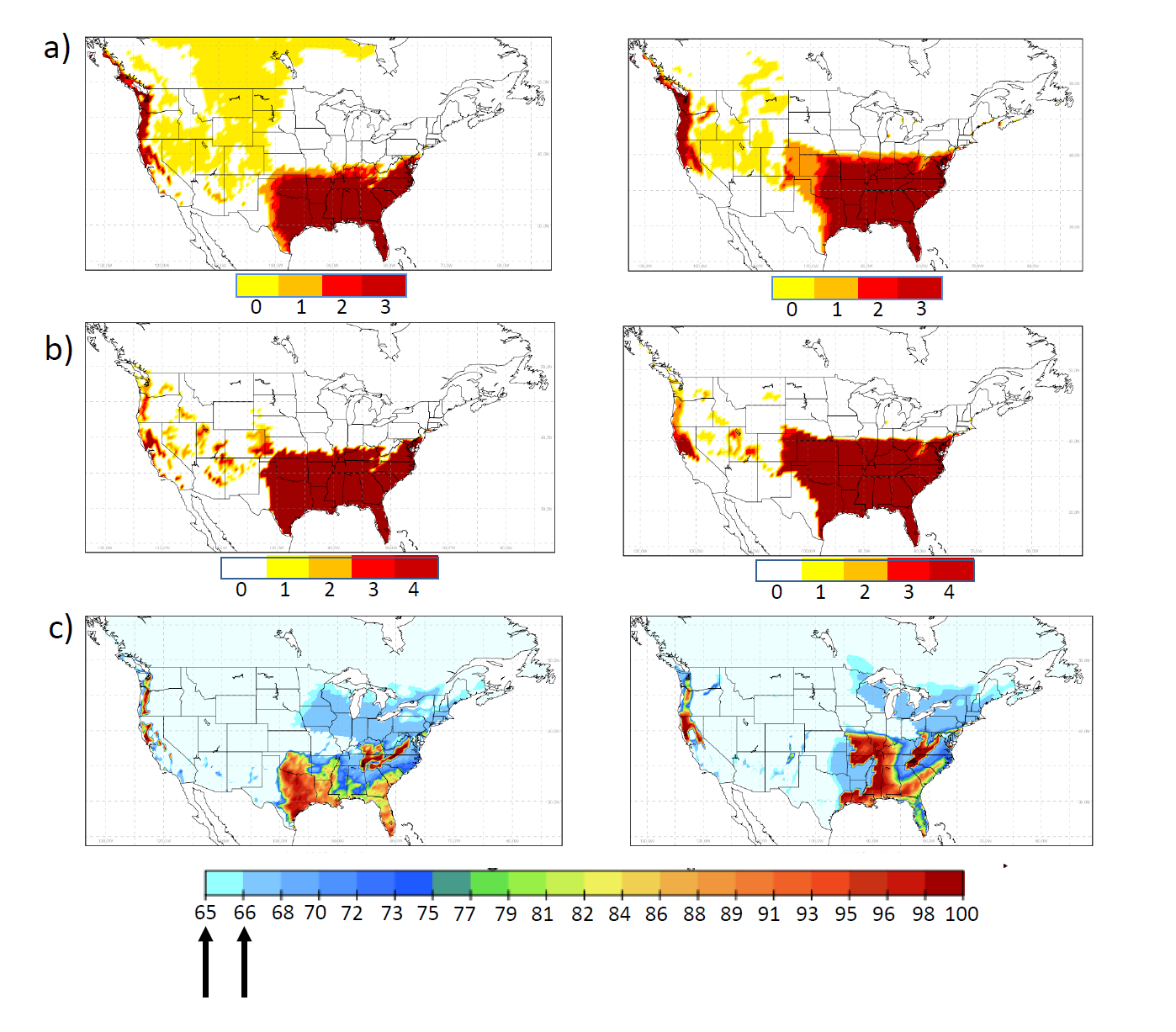
Figure 2: Future climate suitability prediction for Ae. albopictus: a, b, c demonstrate suitability maps of OW, OWAT and SIG indicators for Ae. albopictus with climate change predictions for 30 year periods. Left column: 2011-2040, middle and right column: 2041-2070 based on emission concentrations (Representative Concentration Pathways, RCP4.5 and 8.5). For OW and OWAT the color scales correspond to the suitability scale for these indicators (low to high suitability shown from white or yellow to orange and red based on temperature and precipitation values). For SIG the arrows indicate the 64.64% and 66.69% suitability cut-off values. Used with permission from Ogden et al. 2014. Parasites and Vectors, 7:532 (15).
Aedes aegypti
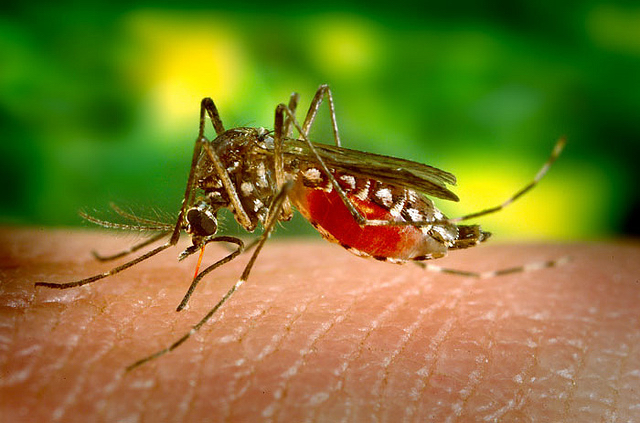
Globally, Aedes aegypti is the primary vector in the transmission of dengue fever and yellow fever. Considered to have originated in Africa, it is believed to have been introduced to the Americas via the transatlantic slave trade (20). Currently, Aedes aegypti is distributed worldwide within tropical and subtropical areas (3) and tends to thrive in areas of human cohabitation, where it uses artificial containers for breeding. The female feeds predominantly on humans, and takes multiple partial blood meals in a single gonotrophic cycle (21), making it an effective vector of pathogens that amplify readily in humans, such as dengue. The geographic range is limited by cold temperatures, with winter temperatures of 10°C thought to be the climatic boundary for survival (22). After nearly being eliminated in at least 18 countries in the American continent, between 1948 and 1962, Aedes aegypti has again reestablished itself in both South and North America, and is linked to the reemergence of dengue (23).
Khormi et al (3) have described a decrease in the geographic range of favourable climatic conditions by 2030, particularly in Brazil, Colombia, Guyana, Paraguay and Venezuela, as well as parts of Southeast Asia. Climate was predicted to continue to be suitable in most of the Pacific and Caribbean islands. In this study, cold stress appeared to be the primary factor limiting Aedes aegypti distribution, as eggs, larvae and adults are vulnerable to freezing temperatures. In both the short-term (2030) and long-term (2070) projections, much of North America remained unsuitable for Aedes aegypti, apart from small areas of Mexico and the Florida peninsula, which were minimally suitable (3). Despite this, recent autochthonous outbreaks of dengue have been documented in Southern Texas (4) and Florida (5,24).
Another study by Eisen et al (25) suggested that climate change has the potential to increase human exposure to the vector Aedes aegypti by increasing the number of annual days where female mosquitos are active and increasing the peak abundance of mosquitos. The researchers suggested that rising temperatures at the margin of geographic range may increase overwinter survival of eggs and promote earlier hatching, extending the season of activity. However, lack of understanding of climatic conditions that allow overwinter survival of eggs and lack of biosurveillance in the cool range margin for Aedes aegypti make it difficult to determine its current geographic range and potential for spread within North America (25).
A model using two greenhouse gas emission scenarios and mean global projections for seven climatic variables representing seasonal and extreme temperatures as well as water availability for 2010-2039 predicted climatic suitability for Aedes aegypti (26). During current climatic conditions, the geographic range of Aedes aegypti was limited from 35°S to 45°N. In this model, regardless of the future climate scenario, geographic range of Aedes aegypti was predicted to increase during the period from 2010-2039. In particular, North-central and North-Eastern United States between 36°N and 48°N were predicted to be climatically suitable for spread of Aedes aegypti. Similar movement to higher latitudes in the north and south was predicted in Europe, Southern Africa and Central Australia (26).
Culex spp.
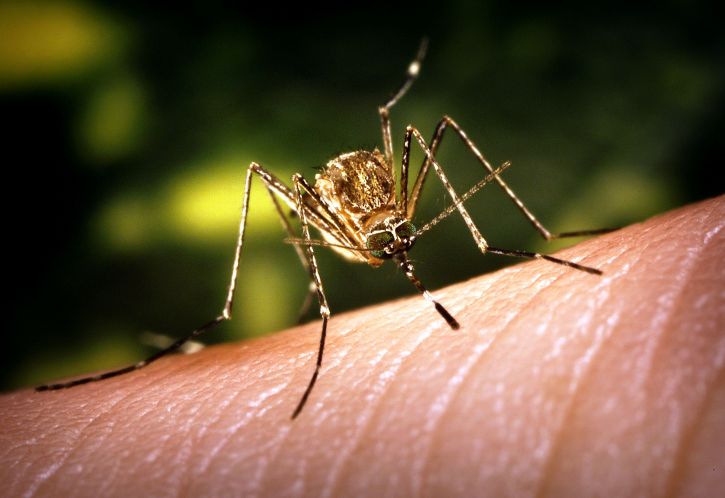
Mosquitoes in the Culex genus (Culex tarsalis, Culex quinquefasciatus, and Culex pipens) are also vectors of concern for North America. Culex tarsalis has been identified as the primary vector of West Nile Virus (WNV, belonging to the flavivirus genus) in Canada. Culex species are geographically dispersed across Canada and the United States. WNV was first introduced into the Western Hemisphere in 1999, where an outbreak occurred in New York City (27). In 2007, 2,215 confirmed clinical cases of WNV infection were reported in Canadians, with 98% occurring in the Prairie Provinces (28). Unlike Aedes albopictus, Culex tarsalis prefers grassland and agriculture areas, breeding in stagnant bodies of water with high organic content (29), found in abundance on the Canadian prairies. Although humans act as a “dead-end” host for West Nile virus, Culex tarsalis feeds on both avian and mammalian hosts, enabling it to act as a “bridging vector” between species. In addition, it is a competent vector of the less common St. Louis and Western Equine Encephalitis viruses (30).
A 2013 Canadian study predicted the effects of climate change on distribution of Culex tarsalis and WNV transmission using three potential emission scenarios in the short (2010-2039), medium (2040-2069) and long (2070-2099) term (29). Two of the three short-term emission scenarios predicted an increase in the WNV infection rate, ranging from a 24%–232% increase in transmission each month. Furthermore, the transmission season was extended (in this case from August until September), a finding that has been seen in studies of Culex quinquefaciatus, a vector of WNV in the Southern United States that inhabits urban environments (31). Long term projections from this study were even more dismal, with up to 27 times the WNV infection rate during peak season in the long-term scenario. The authors also predicted a more northern expansion of the range of Culex tarsalis. Other studies have demonstrated increased reports of WNV in British Columbia associated with elevated summer temperatures, and projected climate change is expected to extend the suitable habitat of Culex pipiens further north in Canada (32).
Resources Available
A number of online resources exist describing the current geographic range of vectors and the incidence of human infection with vector-borne diseases. The Public Health Agency of Canada conducts surveillance of cases of WNV and other vector-borne diseases, which can be accessed at http://healthycanadians.gc.ca (28). The U.S. Geological Survey provides an interactive map of human disease cases reported to CDC ArboNET that can be found at: http://diseasemaps.usgs.gov/mapviewer (33). Walter Reed Biosystematics Unit’s VectorMap (http://vectormap.si.edu) is an interactive portal that provides disease maps and distribution models for a number of arthropod disease vector species (34). These resources, however, are limited in that they represent convenience sampling, rather than systematic surveys.
Relevance for Public Health in Canada
Collectively, the current literature suggests that climate change will result in an increase in the geographic range suitable for mosquito vectors. While there is limited literature describing the anticipated distribution in Canada, the trend is towards a northward expansion in North America, which may be exacerbated in urban areas by the relative refuge offered from climatic extremes. The expanding range of the more promiscuous vector (both in terms of viruses and animal hosts) Aedes albopictus is of paramount importance and relatively short-term concern. Furthermore, increasing trends in trade and travel will make awareness of shifts in geographic distribution of vector-borne disease essential for public health practitioners. Increased awareness of the effects of climate change on transmission of vector-borne disease will help to inform proactive public health strategy.
Table 2: Arboviruses causing disease in the Americas (35)
Disease | Genus | Vector | Host | Geographic distribution |
Chikungunya virus | Alphavirus | Aedes aegypti; Aedes albopictus | Humans | United States, Central & South America, Asia, Pacific Islands, parts of Africa |
Dengue virus | Flavivirus | Aedes aegypti; Aedes albopictus | Humans and other mammals, | Central & South America, Asia, Pacific Islands, parts of Africa |
Eastern Equine Encephalitis | Alphavirus | Aedes spp., Culex spp., Coquillettida spp | Birds; Horses and Humans are incompetent hosts | Freshwater hardwood swamps in the Atlantic, Gulf coast states, Great Lakes |
LaCrosse Encephalitis | Bunyavirus (California serogroup; includes California encephalitis virus, Jamestown Canyon virus, Snowshoe hare virus, Trivitattus virus) | Aedes triseratus (Eastern Treehole mosquito) | Small mammals; Humans are incompetent hosts | Upper mid-western United States |
St. Louis Encephalitis | Flavivirus | Culex pipiens; Culex quinquefasciatus | Birds; Humans and domestic mammals are incompetent hosts | Canada to Argentina; most cases occurring in the United States |
West Nile virus | Flavivirus | Culex spp | Birds; Humans & other mammals are incompetent hosts | Canada, United States |
Yellow fever | Flavivirus | Aedes aegypti; Aedes albopictus (Asian tiger mosquito) | Human and non-human primates | Central Africa, South America |
Zika virus | Flavivirus | Aedes aegypti; Aedes albopictus | Humans | Africa, Southeast Asia, Pacific Islands, Central & South America |
Adapted from: CDC
References
(1) Ogden NH, St-Onge L, Barker IK, Brazeau S, Bigras-Poulin M, Charron DF, et al. Risk maps for range expansion of the Lyme disease vector, Ixodes scapularis, in Canada now and with climate change. Int J Health Geogr 2008 May 22;7:24-072X-7-24.
(2) Solomon S, Intergovernmental Panel on Climate Change, Intergovernmental Panel on Climate Change. Working Group I. Climate change 2007: The physical science basis: Contribution of Working Group I to the Fourth Assessment Report of the Intergovernmental Panel on Climate Change. Cambridge; New York: Cambridge University Press; 2007.
(3) Khormi HM, Kumar L. Climate change and the potential global distribution of Aedes aegypti: Spatial modelling using GIS and CLIMEX. Geospat Health 2014 May;8(2):405-415.
(4) Brunkard JM, Robles Lopez JL, Ramirez J, Cifuentes E, Rothenberg SJ, Hunsperger EA, et al. Dengue fever seroprevalence and risk factors, Texas-Mexico border, 2004. Emerg Infect Dis 2007 Oct;13(10):1477-1483.
(5) Adalja AA, Sell TK, Bouri N, Franco C. Lessons learned during dengue outbreaks in the United States, 2001-2011. Emerg Infect Dis 2012 Apr;18(4):608-614.
(6) Lazear HM, Stringer EM, de Silva AM. The emerging Zika virus epidemic in the Americas: Research priorities. JAMA 2016 May 10;315(18):1945-1946.
(7) Benedict MQ, Levine RS, Hawley WA, Lounibos LP. Spread of the tiger: Global risk of invasion by the mosquito Aedes albopictus. Vector Borne Zoonotic Dis 2007 Spring;7(1):76-85.
(8) Medlock JM, Hansford KM, Schaffner F, Versteirt V, Hendrickx G, Zeller H, et al. A review of the invasive mosquitoes in Europe: Ecology, public health risks, and control options. Vector Borne Zoonotic Dis 2012 Jun;12(6):435-447.
(9) Grandadam M, Caro V, Plumet S, Thiberge JM, Souares Y, Failloux AB, et al. Chikungunya virus, southeastern France. Emerg Infect Dis 2011 May;17(5):910-913.
(10) Rezza G, Nicoletti L, Angelini R, Romi R, Finarelli AC, Panning M, et al. Infection with chikungunya virus in Italy: an outbreak in a temperate region. Lancet 2007 Dec 1;370(9602):1840-1846.
(11) World Health Organization. Chikungunya. 2016; Available at: http://www.who.int/mediacentre/factsheets/fs327/en/. Accessed May, 2016.
(12) Vezzani D. Review: Artificial container-breeding mosquitoes and cemeteries: A perfect match. Tropl Med & Int Health 2007;12(2):299-313.
(13) Waldock J, Parham PE, Lelieveld J, Christophides GK. Climate and human health: The impact of climate change on vector-borne diseases, Paphos, Cyprus (17-19 October 2012). Pathog Glob Health 2013 Dec;107(8):387-392.
(14) Rochlin I, Ninivaggi DV, Hutchinson ML, Farajollahi A. Climate change and range expansion of the Asian tiger mosquito (Aedes albopictus) in Northeastern USA: Implications for public health practitioners. PLoS One 2013;8(4):e60874.
(15) Ogden NH, Milka R, Caminade C, Gachon P. Recent and projected future climatic suitability of North America for the Asian tiger mosquito Aedes albopictus. Parasit Vectors 2014 Dec 2;7:532-014-0532-4.
(16) Fauci AS, Morens DM. Zika Virus in the Americas–Yet Another Arbovirus Threat. N Engl J Med 2016 Feb 18;374(7):601-604.
(17) Bonizzoni M, Gasperi G, Chen X, James AA. The invasive mosquito species Aedes albopictus: Current knowledge and future perspectives. Trends Parasitol 2013 Sep;29(9):460-468.
(18) Campbell LP, Luther C, Moo-Llanes D, Ramsey JM, Danis-Lozano R, Peterson AT. Climate change influences on global distributions of dengue and chikungunya virus vectors. Philos Trans R Soc Lond B Biol Sci 2015 Apr 5;370(1665):10.1098/rstb.2014.0135.
(19) Proestos Y, Christophides GK, Erguler K, Tanarhte M, Waldock J, Lelieveld J. Present and future projections of habitat suitability of the Asian tiger mosquito, a vector of viral pathogens, from global climate simulation. Philos Trans R Soc Lond B Biol Sci 2015 Apr 5;370(1665):10.1098/rstb.2013.0554.
(20) Eisen L, Moore CG. Aedes (Stegomyia) aegypti in the continental United States: A vector at the cool margin of its geographic range. J Med Entomol 2013 May;50(3):467-478.
(21) Scott TW, Chow E, Strickman D, Kittayapong P, Wirtz RA, Lorenz LH, et al. Blood-feeding patterns of Aedes aegypti (Diptera: Culicidae) collected in a rural Thai village. J Med Entomol 1993 Sep;30(5):922-927.
(22) Hopp MJ, Foley JA. Global-scale relationships between climate and the dengue fever vector, Aedes aegypti. Clim Change 2001;48(2):441-463.
(23) Brathwaite Dick O, San Martin JL, Montoya RH, del Diego J, Zambrano B, Dayan GH. The history of dengue outbreaks in the Americas. Am J Trop Med Hyg 2012 Oct;87(4):584-593.
(24) Radke EG, Gregory CJ, Kintziger KW, Sauber-Schatz EK, Hunsperger EA, Gallagher GR, et al. Dengue outbreak in Key West, Florida, USA, 2009. Emerg Infect Dis 2012 Jan;18(1):135-137.
(25) Eisen L, Monaghan AJ, Lozano-Fuentes S, Steinhoff DF, Hayden MH, Bieringer PE. The impact of temperature on the bionomics of Aedes (Stegomyia) aegypti, with special reference to the cool geographic range margins. J Med Entomol 2014 May;51(3):496-516.
(26) Capinha C, Rocha J, Sousa CA. Macroclimate determines the global range limit of Aedes aegypti. Ecohealth 2014 Sep;11(3):420-428.
(27) Nash D, Mostashari F, Fine A, Miller J, O’Leary D, Murray K, et al. The outbreak of West Nile virus infection in the New York City area in 1999. N Engl J Med 2001 Jun 14;344(24):1807-1814.
(28) Public Health Agency of Canada. Surveillance of the West Nile virus. 2016; Available at: http://healthycanadians.gc.ca/diseases-conditions-maladies-affections/disease-maladie/west-nile-nil-occidental/surveillance-eng.php. Accessed 07/12, 2016.
(29) Chen CC, Jenkins E, Epp T, Waldner C, Curry PS, Soos C. Climate change and West Nile virus in a highly endemic region of North America. Int J Environ Res Public Health 2013 Jul 22;10(7):3052-3071.
(30) Hongoh V, Berrang-Ford L, Ogden NH, Lindsay R, Scott ME, Artsob H. A review of environmental determinants and risk factors for avian-associated mosquito arboviruses in Canada. Biodiversity 2009 09/01;10(2-3):83-91.
(31) Morin CW, Comrie AC. Regional and seasonal response of a West Nile virus vector to climate change. Proc Natl Acad Sci U S A 2013 Sep 24;110(39):15620-15625.
(32) Hongoh V, Berrang-Ford L, Scott ME, Lindsay LR. Expanding geographical distribution of the mosquito, Culex pipiens, in Canada under climate change. Appl Geogr 2012 4;33:53-62.
(33) US Geological Survey. West Nile Virus Human Provisional 2015 Data. 2016; Available at: http://diseasemaps.usgs.gov/mapviewer/. Accessed 07/12, 2016.
(34) The Walter Reed Biosystematics Unit. Vector Map: Know the vector, know the threat. 2016; Available at: http://vectormap.si.edu/. Accessed 07/12, 2016.
(35) Centers for Disease Control and Prevention (CDC). Division of Vector-Borne Diseases (VBD). 2016; Available at: http://www.cdc.gov/ncezid/dvbd/. Accessed 07/12, 2016.