CMAJ November 28, 2022 194 (46) E1560-E1567
Listen to our conversation with Jesse Knight about this research on our mod4PH podcast.
Introduction
The outbreaks of mpox virus (MPXV) in Canada disproportionately affected communities which included gay and bisexual individuals and other men who have sex with men (GBMSM). Shortly after the initial cases of MPXV were reported, a third-generation smallpox vaccine (Imvamune®) was deployed to reduce the risk of infection and severe disease. The allocation of a limited supply of MPXV vaccines presented a challenge to public health in the first few weeks of the outbreak. Previous work has demonstrated that prioritizing a limited supply of vaccines to sub-populations experiencing disproportionately higher risk of transmission can reduce the number of infections overall.
This study explored how a fixed supply of MPXV vaccine can be optimally allocated between 2 partially connected networks (cities) in Ontario under different epidemic conditions. The size of the networks, epidemic potential (reproductive number R0), imported cases (seed cases), and the connectedness of the two hypothetical cities were varied in the model to identify optimal vaccine allocation strategies. The goal of this study was to produce generalizable insights into MPXV vaccine prioritization, which can be applied by policy makers across diverse and dynamic epidemic contexts across Canada and beyond.
Methods
A deterministic compartmental model of MPXV transmission which captured both sexual and non-sexual transmission was developed. The model represented 2 partially connected GBMSM communities (in cities A and B) with a combined total size of 100,000. The compartments represented 6 health states: susceptible (S), vaccinated (V), exposed (E), infectious (I), isolating (H) and recovered (R) (Figure 1a). Each city was further stratified by lower and higher sexual risk groups (Figure 1b). The model was parameterized using prior data on GBMSM sexual networks and emerging MPXV epidemiological data. The model was calibrated to produce city-specific reproductive numbers (R0) ranging from 1 to 2 through varying the average numbers of sexual partners among the higher risk group, assuming no between-city mixing.
Possible exposures in the model began up to 21 days prior to the first reported case in Ontario (May 20, 2022), and pre-exposure prophylaxis vaccination began June 12, 2022 (45 days later), with an assumed 5000 available doses. All simulations were initiated with 10 imported seed cases in the higher risk groups. Seed cases were distributed across the exposed, infectious, and isolating stages based on the mean duration of each stage. Modelled distribution of 5000 vaccine doses over 30 days started 45 days after the initial cases were imported, and were imperfectly prioritized to the higher risk groups with 90% sensitivity (4500 doses to the higher risk group, 500 doses to the lower risk group).
Figure 1. Model structure
(a) S: susceptible; V: vaccinated; E: exposed; I: infectious; H: isolating; R: recovered. (b) Risk: risk of mpox virus infection or transmission, defined by numbers of sexual partners (definitions details in Appendix 1). Arrow opacity is qualitatively related to the chance of sexual contact formation from any group to another (higher opacity reflects greater chance of contact). See Appendix 1 Section A (model details) for rate definitions.
The optimal vaccine allocation between cities A and B was explored over a range of epidemic conditions and was defined as that which resulted in the fewest cumulative infections by day 90 in both cities. As one illustrative example, two strategies for vaccine allocation by city were examined: a) proportional to population size and b) “optimal” (as defined above). For this example, epidemic conditions used in the model were as follows:
- Model parameters selected to be representative of a Toronto-like city (city A, GBMSM population = 80,000) and a medium-sized Ontario city (city B, GBMSM population = 20,000), and 10% sexual/social network connectivity.
- City A with R0 = 2.0 and City B with R0 = 1.5, to reflect differences in sexual network density as suggested by the prevalence of sexually transmitted infections across Ontario cities.
- 100% of imported seed cases initiated in city A to reflect observed early MPXV case distribution in Ontario.
The optimal vaccine allocation between cities A and B for a combination of varied conditions was also examined in an uncertainty analysis. The varied epidemic conditions explored in the model were:
- Relative size of city: size of city A versus city B varied from 1/4–4 times.
- Relative epidemic potential (R0) of city A varied from 1–2 (city B fixed at R0 = 1.5).
- Between-city mixing varied from 0–30% of all contacts formed randomly between cities.
- Fraction of imported seed cases in city A versus B varied from 0–100%.
The model and scenarios were implemented in R, with code available online.
Results
The the illustrative example, the model showed that allocating all 5000 vaccine doses to city A resulted in the fewest infections in both cities combined by day 90 (550 infections), while proportional vaccine allocation resulted in 615 infections. No vaccination yielded 1020 infections. In this scenario, optimal vaccine allocation (100% of vaccines to city A) resulted in an exponential increase in infection incidence in city B in the short term, but still resulted in the greatest number of infections averted.
Uncertainty analysis indicated that in all epidemic conditions explored, the relative epidemic potential (R0), distribution of seed cases, and city size were strong determinants of optimal vaccine allocation (Figure 2). A larger city with a higher R0 and the majority of seed cases should be prioritized in vaccine allocation (blue and red corners in Figure 2 panels). Scenarios which resulted in shared vaccine allocation between the two cities (to varying degrees) as the optimal strategy included:
- The smaller city having higher R0 and the majority of seed cases (yellow/green-yellow triangles in Figure 2 a, d, g, c, f, i) due to the ability to vaccinate all higher risk individuals in the smaller city with the available doses.
- Cities with most/all seed cases but a lower R0, or few/no seed cases but a higher R0 (diagonal yellow segments in Figure 2).
Figure 2. Optimal vaccine allocation between 2 cities under different epidemic conditions
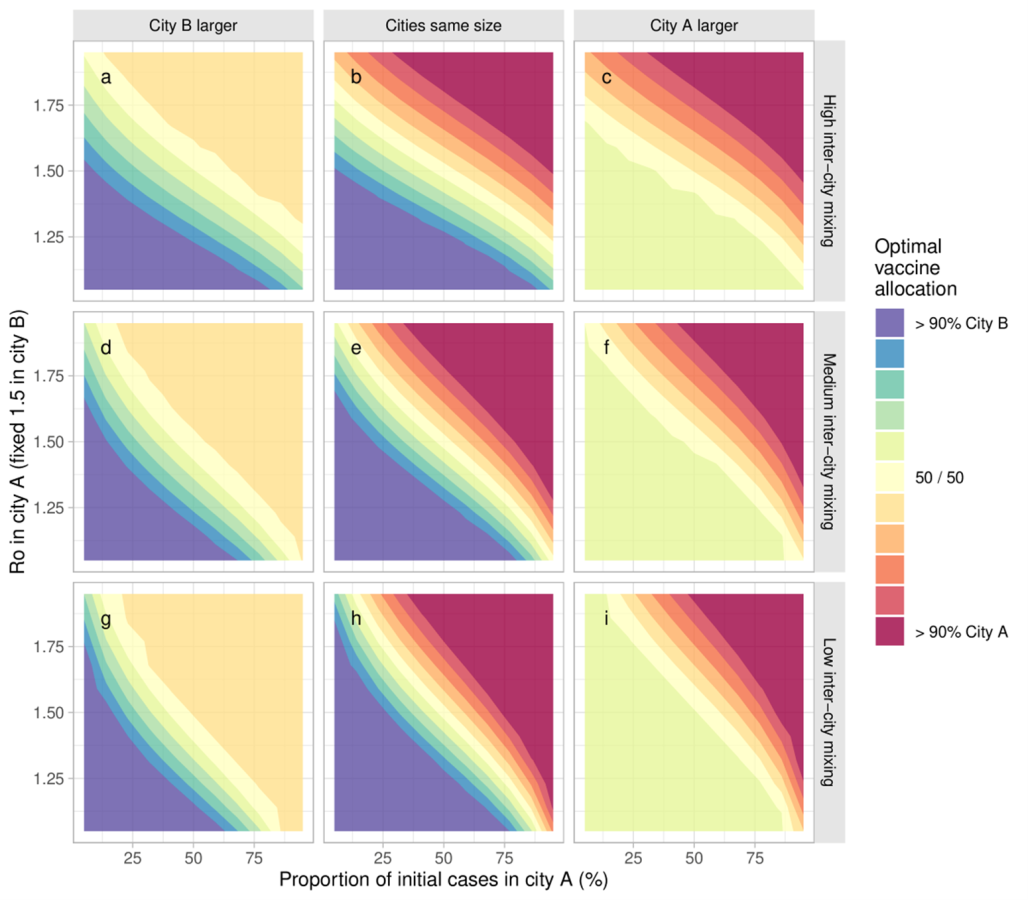
R0 in city A varies via the sexual activity among the higher risk group in city A. Optimal vaccine allocation is defined as fewest cumulative infections by day 90. The larger city is 3 times the size of the other city. εc = 0.8,0.9,0.95 was used for high, medium and low between-city mixing, respectively.
Finally, increased levels of mixing between the two cities reduced the influence of the distribution of initial seed cases and increased the influence of R0 on optimal vaccine allocation to each city. This is shown in the more vertical gradient in panels a, b, c (high inter-city mixing) compared to the relatively more horizontal gradient in panels g, h, i (low inter-city mixing) (Figure 2).
Discussion, limitations, and conclusion
This study sought to explore how different epidemic conditions could affect optimal allocation of a limited supply of MPXV vaccines across 2 partially connected networks. The model outcomes showed that prioritizing a larger network with more initial infections and higher epidemic potential (R0) in the vaccination strategy results in a greater number of infections averted. These results are consistent with previous studies which showed that prioritizing a limited vaccine supply or resources to sub-populations experiencing disproportionate risk generally yields the greatest benefits for the overall population. This study also highlights that optimizing vaccine allocation requires a better understanding of the different drivers of epidemic potential which result in disproportionate risk between populations.
While this study used a simple deterministic compartmental model of 2 partially connected networks with a fixed vaccine rollout approach, it illustrates the importance of measuring outcomes for interconnected populations. Additional data on transmission networks, service availability, and vaccine uptake in different jurisdictions and communities may provide additional insights for optimizing vaccine allocation. However, the general principles and findings of this study are expected to hold across health units, provinces, or even countries. Improved understanding of the epidemic potential between different populations can support resource prioritization to eliminate existing disparities in healthcare access and outcomes.